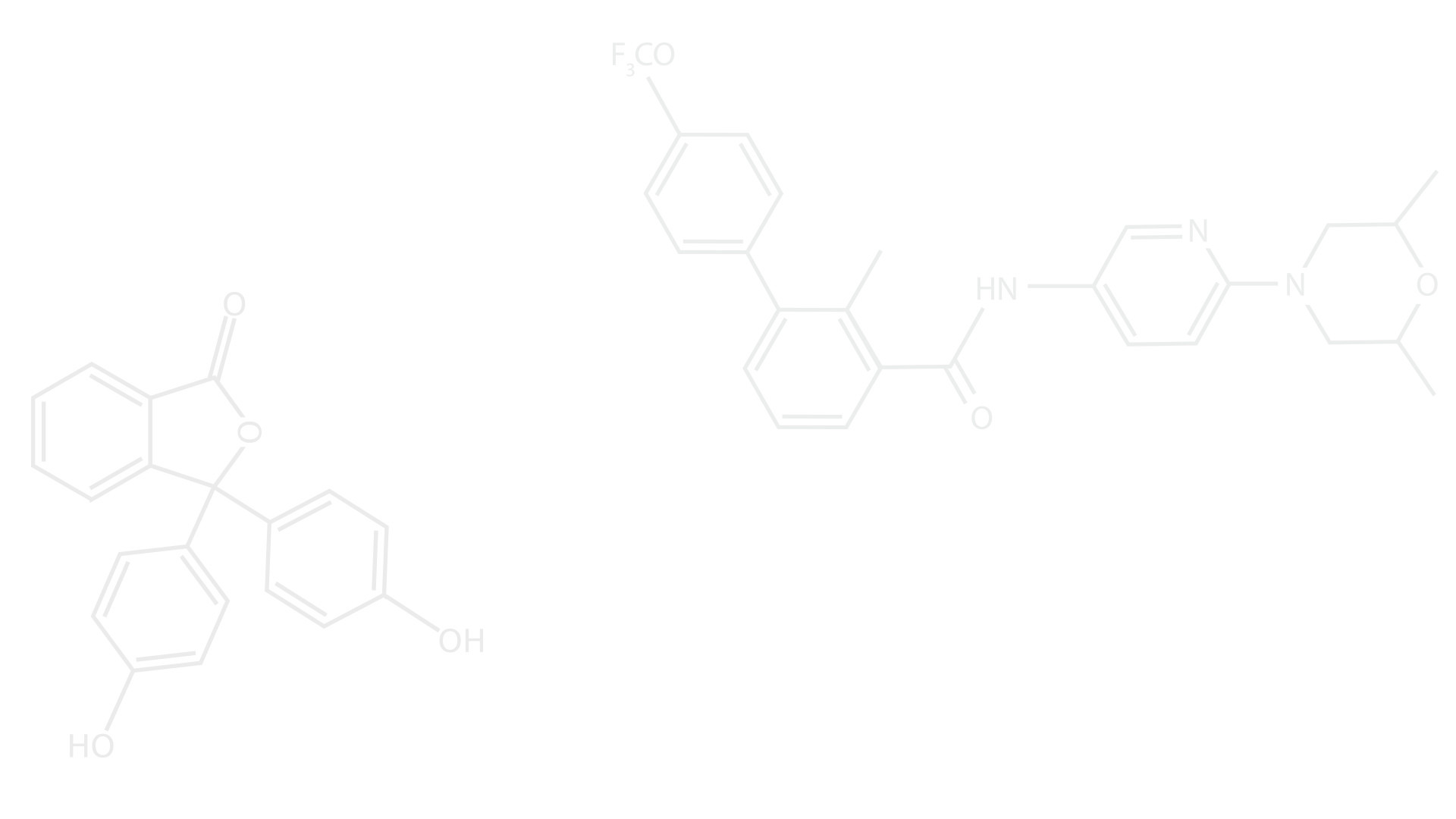
Preprint Club
A cross-institutional Journal Club Initiative
Monocyte control of organismal energy homeostasis
Martins*, Blankehaus* et al. (BioRxiv) DOI: 10.1101/2025.02.20.639373
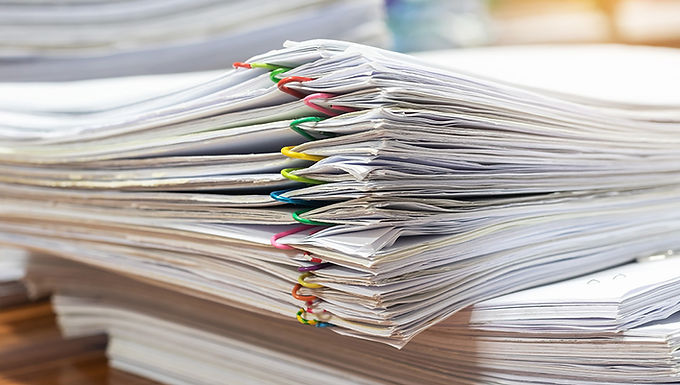
Keywords
Iron metabolism
Myeloid cells
Monocytes
Energy metabolism
Ferritin
Main Findings
Systemic and cellular iron metabolism is essential to maintain organismal homeostasis. As such cellular iron is a key nutrient controlling several biological processes including energy production, redox balances, gene and epigenetic regulation. Unsurprisingly, aberrant iron levels due to nutrient deficiencies or infections, can have far reaching impact on organ homeostasis and thus alter systemic responses.
Since iron is typically maintained through a delicate balance of iron uptake, storage and release, perturbances of this system is often associated with far reaching consequences to organismal health. In a previous study, Blankehaus et al. found that the post-natal loss of the ferritin heavy chain (Fth), a key protein that allows for safe intracellular storage of iron, disrupts energy metabolism and results in multi-organ failure and premature death (1). In a follow-up study, Martins and Blankehaus et al. investigate the cellular cause of this lethality using a series of bone marrow transplantation models.
Lethality of Fth deletion was rescued when mice received wild-type bone marrow, indicating a crucial function of Fthexpression in immune cells. To narrow down the necessary subset, the authors transplanted bone marrow with a myeloid cell-specific deletion of Fth, which was unable to prevent Fth lethality. This suggested that myeloid cell-specific Fth expression is required to prevent the lethal phenotype observed in this mouse model. In line with this, transplantation of wild-type bone marrow also rescued several aspects of systemic iron levels (including transferrin levels and transferrin saturation), as well as disruption of systemic energy metabolism (measured through total energy expenditures and body heat production). Moreover, wild-type bone marrow restored systemic redox homeostasis and cardiac function. All of these changes appeared myeloid dependent, since transplantation of bone marrow carrying the myeloid-specific deletion of Fth was unable to rescue these effects lastingly.
To narrow down the myeloid population responsible for this restoration of the phenotype, the authors analysed presence of myeloid populations in their transplanted animals. They found that mice reconstituted with an Fth-deficient bone marrow had a near complete absence of infiltrating monocyte-derived macrophages in several tissues. These populations were restored upon transplantation of wild-type bone marrow. To further validate that circulating monocytes may be a crucial component to the restoration of this phenotype the authors used parabiosis experiments. Connecting the circulatory system of wild-type mice with Fth-deficient mice, was sufficient to rescue the lethal phenotype indicating that some circulating factors or cells may mediate this effect. Additional experiments, such as CX3CR1-specific Fth deletion as well as monocyte transfers indicate that monocyte-derived macrophages may represent an important cell type regulating Fth-deficiency associated systemic changes.
Lastly, the authors aimed to identify the mechanism through which wild-type bone marrow transplantation may rescue the changes in systemic metabolism and lethality. The phenotype seemed to be independent of myeloid specific uptake or release of iron since both the deletion of Transferrin receptor (Tfrc) as well as Ferroportin (Slc40a1) was dispensable for lethality. Using a series of experiments, the authors excluded two further hypotheses involving either the direct transfer of ferritin from immune cells to parenchymal cells using V5-tagged Fth as well as the direct transfer of mitochondria using a mitochondrial reporter bone marrow. Nevertheless, the authors observed that tissues from mice that were reconstituted with wild-type bone marrow showed a marked improvement in expression of mitochondrial genes and proteins. Moreover, the authors found that myeloid specific mitochondrial biogenesis (through transplantation of bone marrow carrying a myeloid-specific deletion of its master transcription factor TFAM) phenocopied the lethality phenotype observed in Fth-deficient myeloid cells, thus indicating that myeloid cells may require functional mitochondrial turnover. In contrast, myeloid-specific loss of components of the electron transport chain (i.e. CIII) still prevented lethality indicating that oxidative phosphorylation in myeloid cells may not be required for the rescue effects.
Limitations
The authors are commended for their substantial efforts in this study and pull together a set of impressive efforts in various transplantation models to delineate the mechanisms. Yet, a key limitation of the study is the identification of the precise mechanism by which transplantation of wild-type bone marrow is able to prevent Fth-associated lethality and changes in energy metabolism. A couple of additional experiments will help to solidify the findings and strengthen the study:
Investigation of erythrocyte lineages (how well are they reconstituted in both their wild-type bone marrow and myeloid-specific Fth specific deletion setting). This may be especially relevant since macrophages take an essential part the turnover of erythrocytes. Does this lead to differences in oxygen availabilities to the organ and shift systemic redox potential?
Cellular iron state. Do the cells “think” they have sufficient iron or not? This could be measured through IRE/IRP iron regulatory system by qPCR, especially in their monocyte population.
Iron-sulfur cluster synthesis is occurring in the mitochondria. Would it be possible that this is changing the in the myeloid cells upon Fth-loss and could this explain the rescue of the phenotype? Does loss of Fth in myeloid cells also lead to a loss of mitochondrial ferritin?
The authors previously showed that deletion of Fth in specific immune cell subsets such as regulatory T cells can have functional impacts on their stability and function. However, the functional impact of Fth knockout in myeloid cells was not characterized here. Do these macrophages still phagocytose, metabolize heme, etc.?
In general, a deeper understanding of the redox state of parenchymal cells as well as glutathione levels may require further investigation to understand the underlying phenotype.
An improvement to the Tfrc knockout experiment would be to confirm that the cells are not taking up iron through Tfrc-independent mechanisms.
Of note, an important piece of information that the authors could consider moving into the main figures is the fact that Fth-deficient bone marrow alone is not sufficient to induce the lethal phenotype (Suppl. Fig 1) indicating the cooperative deletion is required between parenchymal cells and myeloid cells.
Significance/Novelty
Macrophages have been shown previously to support tissue homeostasis through regulating iron metabolism. However, the preprint from Martins and Blankehaus et al. demonstrates a role for monocytes in the homeostasis of systemic energy metabolism through a critical supportive role via iron metabolism. Interestingly, their role appears independent of previously described mechanisms in macrophages. Thus, uncovering the critical support mechanism in this model should be an exciting contribution to our understanding of immunology and energy homeostasis.
Credit
Reviewed by Felix Clemens Richter and Kelsey Voss as part of a cross-institutional journal club between the Max-Delbrück Center Berlin, the Ragon Institute Boston (Mass General, MIT, Harvard), the University of Virginia, the Medical University of Vienna and other life science institutes in Vienna.
The author declares no conflict of interests in relation to their involvement in the review.